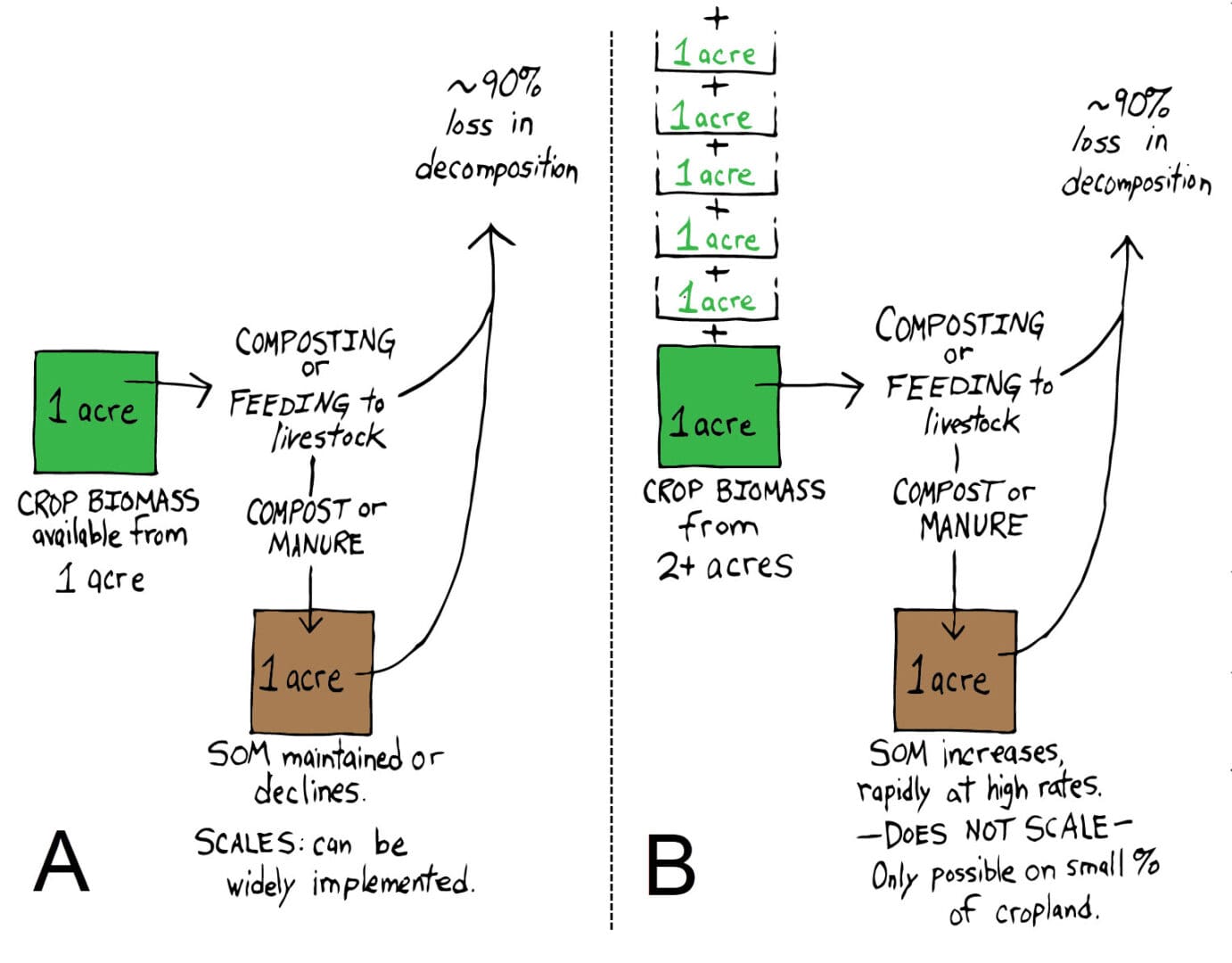
Putting Numbers to the Difficult Task of Increasing Soil Organic Matter
October 25, 2023
Author: Andy McGuire
You may know that it is difficult to increase soil organic matter, but how difficult is it, with numbers? First, your crop harvest removes up to 50% of the biomass grown. Then, about 90% of the remaining crop biomass is decomposed by soil organisms leaving only 10% contributing to soil organic matter. You also have to account for the annual 1-5% losses of existing soil organic matter. Using these and other estimates, let’s do some rough calculations so you know what to expect. The task is difficult, but the math is easy, I promise.
A matter of Inputs and Losses
Here is the basic equation (Janzen et al. 2022).
ΔSOM = Biomassin - Lossesout
The change in soil organic matter (SOM), either positive or negative is equal to the crop biomass inputs minus the losses due to decomposition of both crop biomass and of existing SOM. This is the case no matter the specific mechanisms leading to SOM formation, roots, dead microbes, etc. (Caruso et al. 2018; Janzen et al. 2022).
The equation tells us the primary limiting factor for soil organic matter is plant production, or Biomassin in the equation above (Fujisaki et al., 2018). Therefore, photosynthesis constrains the upper limit of SOM levels.
“Since all soil C is derived from photosynthesis, the amount of net primary productivity (NPP) applied to soil must present the ultimate limit on additional C sequestration.” Janzen et al., 2022
For these ballpark calculations, the results will be roughly equal whether using carbon (C) or total dry biomass. This works because C is about half of plant biomass and organic soil-C is about half of soil organic matter. Also, I am assuming all the weights are dry weights when some of them (yield to residue values) include low moisture amounts. And I am not going to cover the nutrients needed to increase SOM. Let’s start with that 90% number.
Feeding your soil takes 90% of added plant biomass
At least 90% of crop biomass inputs added to soils is consumed by soil organisms (mostly microbes) and goes back to the atmosphere as CO2 (Berthelin et al. 2022; see also Janzen et al. 2022). Put another way, for every 10 lb., tons, or kg of crop biomass going into your soil, you get 1 lb., ton, or kg of soil organic matter, a 10:1 ratio. About half of this loss occurs in the first year, increasing to 80% after 7 years, and reaching the 90% loss after 30 years, but it can happen much faster depending on several factors. Warmer, wetter conditions, and soil disturbance all increase the speed of these losses.
Berthelin et al. (2022) believe this is a conservative estimate, most relevant when the soil has a large capacity for holding organic matter. As SOM levels build, it becomes increasingly difficult to increase SOM, thus requiring more than the 10:1 ratio.
A modest increase of soil organic matter
Let’s say you want to increase your soil’s organic matter from 1.0% to 1.1% in one year, or an increase of one percentage point (to 2%) over 10 years. Not a bad goal. If we just look at the surface 6”, a 1% SOM soil has 20,000 lb. of SOM per acre:
One acre of soil, 6” deep, weighs about 2,000,000 lb. (acre furrow slice).
1% SOM: 2,000,000 × 0.01 = 20,000 lb. of SOM
Our 0.1 percentage point increase is then about 2000 lb.:
1.1% SOM: 2,000,000 × 0.011 = 22,000 lb. of SOM
22,000 - 20,000 = 2000 lb.
Unfortunately, this is not all that is needed. We also need to replace the SOM that will decompose during the year.
Maintaining existing soil organic matter
The continuous loss of existing organic matter provides the benefits of mineralized nutrients (the nitrogen credit from SOM), and food for soil organisms (Janzen 2006), but to sustain these benefits, the loss must be replaced by new SOM. The size of this loss is harder to estimate as it depends on both location – soil and climate – and on practices – tillage and irrigation. With minimal erosion, annual loss rates range from 1-5% of total SOM (Magdoff and Weil, 2004).
Let’s look at a best-case scenario: you live in a cooler Northerly state, practice no-till on a soil with a good amount of clay in it, resulting in only 1% loss.
Annual Loss of SOM: 20,000 × 0.01 = 200 lb. SOM lost
But wait, that was for a soil with 1% SOM. Given the conditions above, you might have 5% SOM, in which case you would need 1000 lb. more. This annual loss factor is why it becomes increasingly difficult to increase SOM as SOM levels increase; the more you have, the more you have to maintain.
We also have to account for the long-term change in SOM as a result of land-use change. This can be positive, as it is here in the Washington’s Columbia Basin where irrigation has increased the plant inputs to the soil which results in higher SOM levels. However, the change is often negative, as where annual crop production replaces native grassland. In this case, the decrease of soil organic matter due to reduced inputs or tillage may continue for decades until an new equilibrium is reached. Because of the change in inputs, other practices such as reduced tillage may only slow the decrease (More on this here). This is not easy to estimate, so I’ll ignore this factor in our calculations and let’s stick with our 1% SOM soil instead of the 5% soil.
So, our total biomass needs for a yearly increase of 0.1 percentage point and to account for yearly loss of SOM is 2200 lb. of soil organic matter:
2000 lb. for the percentage point increase of 0.1
200 lb. to replace decomposing organic matter.
The next question is, how much plant biomass will this require and will your crops provide it?
Contribution of Roots and Root Exudates
Because they are much more difficult to measure, estimates for root biomass, including exudates, and for the rate of conversion of root biomass to SOM vary widely. There is more uncertainty with these numbers. However, what is clear is that in annual crops, root biomass is a small percentage of the shoot biomass. Here I used a Root:Shoot ratio of 0.21 (Pausch and Kuzyakov, 2018). It is also clear that the conversion of root biomass to SOM is much higher than for shoot biomass. Fujisaki et al. (2018) find a range of 1.5-3 times that of the shoot biomass conversion. I will use the 3x or 30% conversion rate.
Crop biomass required
Using the 10:1 ratio for aboveground biomass and the root contribution given above, the crop aboveground (shoot) biomass needed to accomplish this is 13,497 lb. or 6.7 tons/ac per year
Shoot biomass × 0.1 + Root biomass x 0.3 = 2,200 lb. SOM increase
Root/shoot = 0.21,so: Root biomass = Shoot biomass × 0.21
(Shoot biomass × 0.1) = (Shoot biomass × 0.21) × 0.3 = 2,200 lb. SOM increase
Shoot biomass = 13,497 lb.
Crop biomass can be estimated by yield and the crop’s harvest index. With a harvest index of 0.5 for both corn and wheat, the weight of aboveground crop residue ≈ grain yield. To get our needed 6.7 ton/ac of residues, we need a wheat yield of 223 bu. or a corn yield of 239 bu. corn crop.
6.7 tons 2000 lb. bu wheat
⎯⎯⎯⎯ x ⎯⎯⎯⎯ x ⎯⎯⎯⎯ = 223 bu./ac
acre ton 60 lb.
6.7 tons 2000 lb. bu wheat
⎯⎯⎯⎯ x ⎯⎯⎯⎯ x ⎯⎯⎯⎯ = 239 bu./ac
acre ton 56 lb.
These are high yields, above the US averages, so achieving this SOM increase using only crop residues will take longer than a year. And these are high residue crops; getting our needed 6.7 tons/ac with lower residue crops will take even longer.
What can be done to make this better? There are two ways to increase crop biomass inputs to the soil:
- Increase total crop biomass production
- Increase the amount of crop biomass production going to the soil (reduce harvest)
What if we use cover crops? They do both.
Cover crops help...a little
Cover crops increase total biomass production, and because they are not harvested, 100% of their biomass is available to build SOM. The problem with cover crops is that they don’t get much prime growing season. For good reasons, cash crops take up most of the best growing season, leaving shorter, less productive growing windows for cover crops. At the high end of cover crop production, a well-managed, fertilized, irrigated mustard cover crop, planted after wheat in August, so it gets some of the productive summer season, will produce ~4.8 dry tons/ac of biomass (shoots and roots).
Using this number, we then only need 1.9 tons/ac for our SOM increase (6.7 – 4.8 = 1.9 tons/ac), which translates to a 63 bu/ac wheat crop or a 67 bu/ac corn crop.
1.9 tons 2000 lb. bu wheat
⎯⎯⎯⎯ x ⎯⎯⎯⎯ x ⎯⎯⎯⎯ = 63 bu./ac
acre ton 60 lb.
1.9 tons 2000 lb. bu wheat
⎯⎯⎯⎯ x ⎯⎯⎯⎯ x ⎯⎯⎯⎯ = 67 bu./ac
acre ton 56 lb.
Now we are getting somewhere, as these yields are feasible in many regions. However, remember this is for a modest SOM increase in a soil with low SOM and low annual losses, a best case scenario. And most cover crops will produce much less than 4 tons/ac. In many regions with higher SOM and higher losses, this would still be out of reach. This is why cover crops, although having many other benefits including the primary one of minimizing erosion, have not been found to be highly effective in increasing soil organic matter levels (see also Chaplot and Smith, 2023). Given their modest biomass production, they are better at helping maintain SOM levels than increasing them.
See Figure 1 for a visual representation of these calculations.
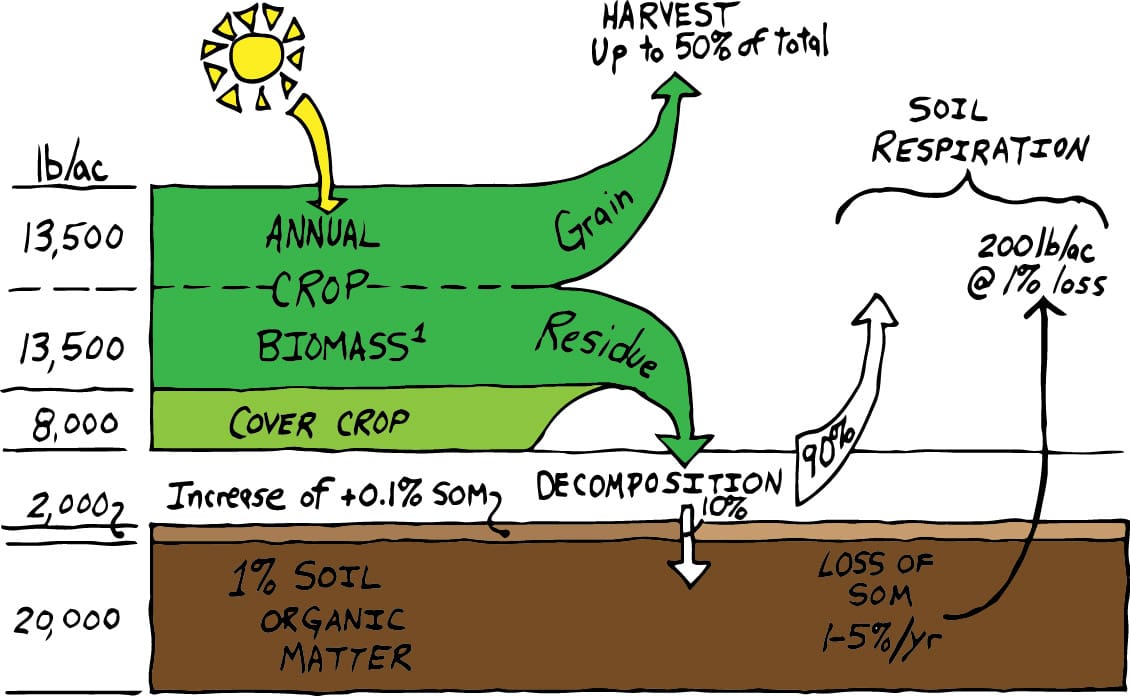
How about manure and compost?
Manure and compost help...but are not the answer
Manure and compost are great tools if you have access to them at the right cost. When applied at high rates, they may seem like magic, but they aren’t:
- While they would seem to get around the 10:1 inputs to stored SOM ratio, it’s an illusion caused by ignoring the source of the organic amendments. If you go back to the crops or plants that became manure or compost, we see that the losses are still there; with manure, inside the livestock, and with compost, in the composting process (Tiquia et al., 2002). From photosynthesis to manure or compost to soil organic matter, the losses are similar, near 90%.
- Their results don’t scale. At the high rates needed to increase SOM, applying manure and compost is a concentration of plant biomass from a large area onto a smaller area. This concentration allows you to produce SOM levels above what the productivity of the land would dictate, but for this reason, it is not sustainable on a large scale. (I have written previously about manure and compost here). Applying manure or compost at the rate that the land receiving them produces biomass would result in SOM gains similar to returning fresh plant biomass.
Figure 2. The use of compost and manure to increase soil health must be viewed in light of the productivity of the land it is applied to. In A, what is applied is equivalent to the land’s productivity, but this will often not increase soil organic matter. On the other hand, in B, the amount applied is equivalent to 2+ acres, and depending on the rate, can increase organic matter rapidly. The latter does not scale.
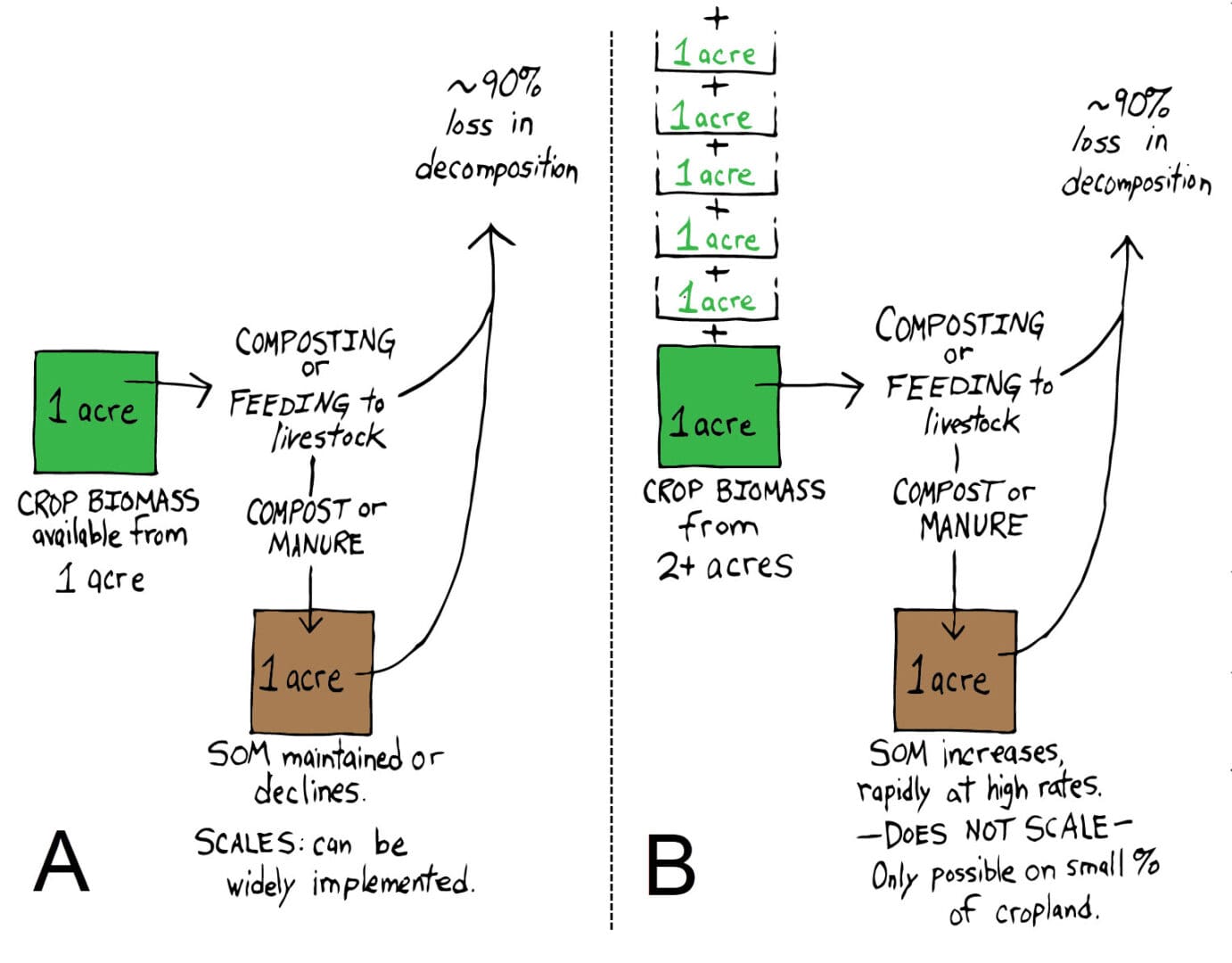
If you are in the enviable position of having access to lots of manure or compost, great. Use it and improve your soils, but we shouldn’t assume that this is an option for more than a small portion of cropland.
What if we grow perennials for grazing?
Perennials and grazing help
Now we are getting somewhere, but at the expense of not growing annual crops. Growing only perennials for grazing, as often found with regenerative agriculture, both increases biomass inputs and decreases SOM losses. Inputs are increased through the longer growing season of perennial forages and by reducing harvest. The harvested meat in grazed livestock removes much less biomass than crop harvest, leaving more to go through the 10:1 decomposition process. Losses are reduced because tillage is eliminated.
Furthermore, perennial produce more root biomass, which as we saw before is more efficiently converted to SOM. While the Root to Shoot ratio of annual crops is around 0.21, for perennial crops, it is can range from 1 to over 3 (Bolinder et al., 2002; Sainju et al., 2017).
The calculations for livestock grazing systems get complicated because there are so many factors, so I will not be doing them. Just know that the 10:1 ratio still applies here for aboveground biomass, but the inputs and losses change, and there are more roots. Perennial hay crops, where almost all the aboveground biomass is removed, are more like annual crops for the purpose of building SOM.
Concentrating SOM at surface helps
An alternative to increasing inputs or decreasing losses is to direct your soil’s organic matter to where it matters most: the surface.
That 0.1 percentage point gain in the top 6” of soil that took 6.7 tons of plant biomass to achieve will only take 3.4 tons/ac in the top 3”, an only 2.2 tons/ac for the top 2” of soil. This makes increasing SOM much more feasible, and the surface is where many of the soil’s important (to agriculture) functions take place. A functioning soil surface reduces wind and water erosion, supports free exchange of air, enhances biological activity, allows seedlings to emerge unhindered and plant roots to proliferate, and perhaps most important, promotes quick water infiltration and movement deep into the soil.
While no-till reduces soil disturbance and therefore reduces losses of SOM, it does not add anything to the input side. Another no-till benefit, however, is allowing SOM to concentrate at the soil surface rather than incorporating residues deeper as do most tillage practices.
Maintaining soil organic matter is becoming more difficult.
The numbers confirm the difficulty of increasing soil organic matter levels. And it is getting worse. As the climate warms, so do soils, resulting in higher losses of SOM every year. It is estimated we will need 50-90% more biomass going into soils just to maintain soil organic matter levels (Riggers et al., 2021). This increase will be needed before we even start to think about increasing SOM levels. Add to this the risk of less frequent but higher intensity storms resulting in more erosion…in many locations, we may struggle to maintain SOM levels, let alone increase them. Even using the best practices.
Energy flow through soil; A silver lining?
At this point, I’d like to give you some positive numbers, but I couldn’t come up with any. However, what if we’re looking at it wrong? Driven by chasing soil health and C sequestration, is our current focus on building up soil organic matter really what matters? We need to remember that the benefits of carbon fixed by crop photosynthesis don’t only come when it becomes long-term SOM. The 90% decomposition rate represents an energy flow through the soil that is also beneficial (Janzen 2015).
“Organic matter is most useful, biologically, when it decays…organic matter is fuel for soil’s biological machinery" Janzen 2006
Soil is not just a bucket for C storage. It is rather a valve controlling the flow of C. Depending on our management, that flow can be increased or decreased, and timing can be adjusted. Given the difficulty of increasing soil organic matter, and climate change will make it increasingly difficult, perhaps we should focus on maintaining current SOM levels and C/energy flow through the soil. That may be all we can hope to do.
References
Berthelin, J., M. Laba, G. Lemaire, D. Powlson, D. Tessier, et al. 2022. Soil carbon sequestration for climate change mitigation: Mineralization kinetics of organic inputs as an overlooked limitation. European Journal of Soil Science 73(1): e13221. doi: 10.1111/ejss.13221.
Bolinder, M.A., D.A. Angers, G. Bélanger, R. Michaud, and M.R. Laverdière. 2002. Root biomass and shoot to root ratios of perennial forage crops in eastern Canada. Can. J. Plant Sci. 82(4): 731–737. doi: 10.4141/P01-139.
Caruso, T., F.T. De Vries, R.D. Bardgett, and J. Lehmann. 2018. Soil organic carbon dynamics matching ecological equilibrium theory. Ecology and Evolution.
Chaplot, V., and P. Smith. 2023. Cover crops do not increase soil organic carbon stocks as much as has been claimed: What is the way forward? Global Change Biology: gcb.16917. doi: 10.1111/gcb.16917.
Fujisaki, K., T. Chevallier, L. Chapuis-Lardy, A. Albrecht, T. Razafimbelo, et al. 2018. Soil carbon stock changes in tropical croplands are mainly driven by carbon inputs: A synthesis. Agriculture, Ecosystems & Environment 259: 147–158. doi: 10.1016/j.agee.2017.12.008.
Janzen, H.H. 2006. The soil carbon dilemma: Shall we hoard it or use it? Soil Biology and Biochemistry 38(3): 419–424. doi: 10.1016/j.soilbio.2005.10.008.
Janzen, H.H. 2015. Beyond carbon sequestration: soil as conduit of solar energy. European Journal of Soil Science 66(1): 19–32. doi: 10.1111/ejss.12194.
Janzen, H.H., K.J. van Groenigen, D.S. Powlson, T. Schwinghamer, and J.W. van Groenigen. 2022. Photosynthetic limits on carbon sequestration in croplands. Geoderma 416: 115810. doi: 10.1016/j.geoderma.2022.115810.
Magdoff, F., and R. Weil. 2004. Soil Organic Matter Management Strategies. Soil Organic Matter in Sustainable Agriculture. CRC Press
Pausch, J., and Y. Kuzyakov. 2018. Carbon input by roots into the soil: Quantification of rhizodeposition from root to ecosystem scale. Global change biology 24(1): 1–12.
Riggers, C., C. Poeplau, A. Don, C. Frühauf, and R. Dechow. 2021. How much carbon input is required to preserve or increase projected soil organic carbon stocks in German croplands under climate change? Plant Soil 460(1): 417–433. doi: 10.1007/s11104-020-04806-8.
Sainju, U.M., B.L. Allen, A.W. Lenssen, and R.P. Ghimire. 2017. Root biomass, root/shoot ratio, and soil water content under perennial grasses with different nitrogen rates. Field Crops Research 210: 183–191. doi: 10.1016/j.fcr.2017.05.029.
Tiquia, S.M., T.L. Richard, and M.S. Honeyman. 2002. Carbon, nutrient, and mass loss during composting. Nutrient Cycling in Agroecosystems 62(1): 15–24. doi: 10.1023/A:1015137922816.
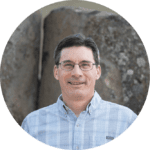
Andy McGuire
Andy is an agronomist working in the Columbia Basin’s irrigated cropping systems. His current focus is on helping farmers build soils, save money, and maintain yields through high residue farming systems and cover cropping.
This article was published by the Washington Soil Health Initiative. For more information, visit wasoilhealth.org. To have these posts delivered straight to your inbox, subscribe to the WaSHI newsletter. To find a soil science technical service provider, visit the Washington State University Extension website or the Washington State Conservation District website.