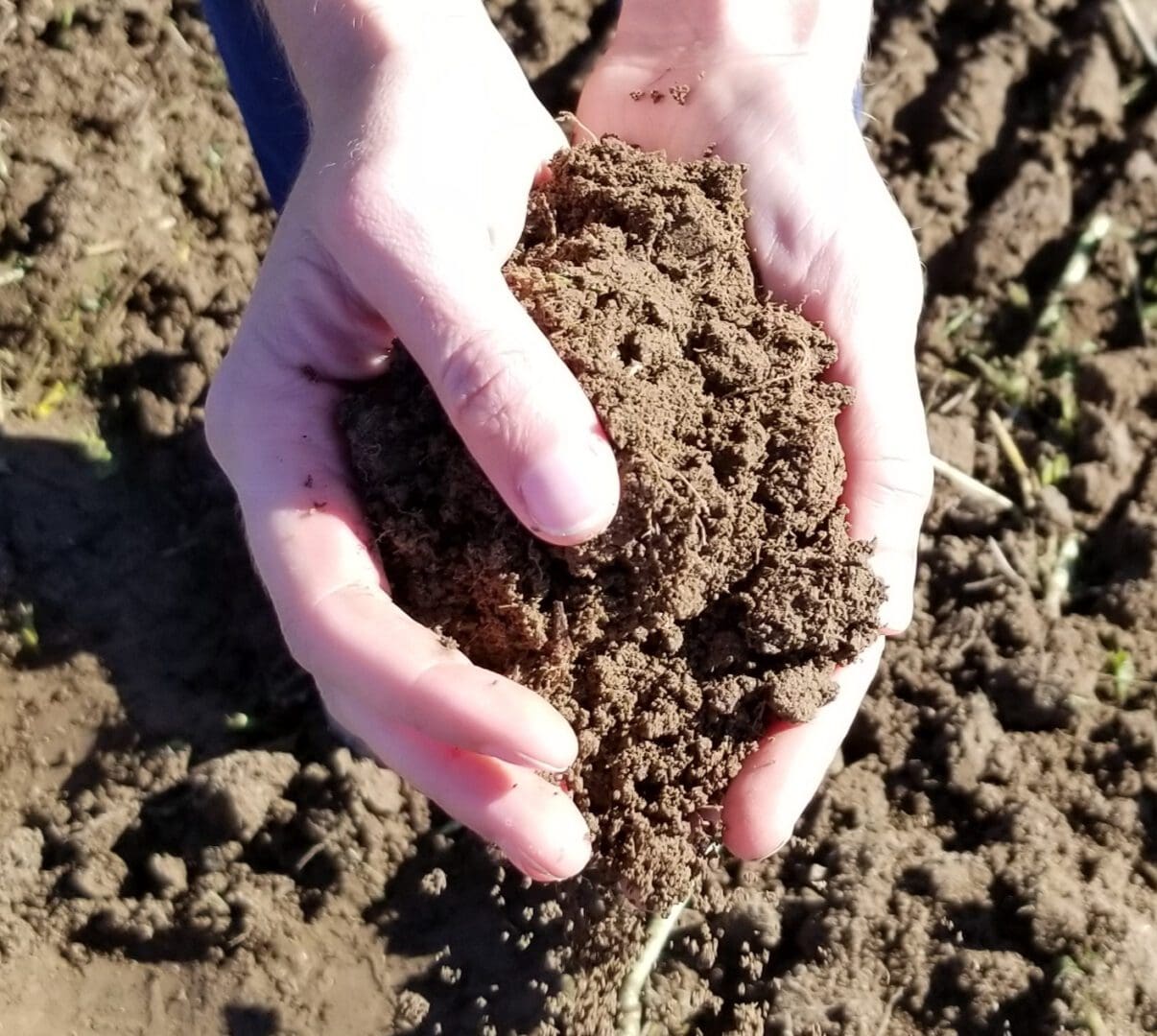
What is “soil health,” anyway?
Soil health is an evolving concept that goes beyond soil quality to emphasize the vital role of soil organisms and their functions, and its indicators, like permanganate-oxidizable carbon and mineralizable C, help us assess soil vitality and guide effective management practices
June 5, 2020
Author: Deirdre Griffin LaHue
Soil health has become a popular topic in agricultural and environmental sectors over the last decade, and soil scientists around the world have rejoiced that others are recognizing the importance of soils to the systems on which our societies rely. However, like any term that becomes a buzz word, we need to keep coming back to reflect on its meaning, to make sure that we are in fact striving to steward healthy soils in a way that makes sense for our agroecosystems. So, what is soil health, anyway?
Some say that soil health is just a new way of referring to soil quality. While many of the concepts and promising management practices are indeed similar, I see soil health as building on soil quality, encompassing a deeper recognition of the importance of the life in the soil, the organisms that drive so many of the functions we associate with high quality soils. Soils are alive, and new analysis tools are allowing us to look more closely at what organisms are there, what they are doing (breaking down organic matter, making nutrients available to plants, building soil structure), and how soil management affects them. In my mind, this is a cornerstone of soil health research.
How do we know if our soils are healthy?
Just as vital signs help give a picture of human health, soil health indicators are being developed to help us tell a healthy soil from an unhealthy one. These indicators include measurements of properties that are chemical (e.g. organic matter content, pH), physical (e.g. soil compaction, water holding capacity), and biological (e.g. microbial biomass or microbially available carbon). Less quantitative tests can also be done quickly and cheaply in the field (Figure 1 A and B). These properties relate to key functions we look for from soils, including supporting plant growth, cycling and storing nutrients and carbon, filtering water, and maintaining these functions in the face of disturbances, like drought, flooding, or disease pressure (resilience).
But just as a 25-year-old man might have different target vitals than a 45-year-old woman, soil types differ in what might be “healthy” values for these indicators. Therefore, there is still a lot of work to do to define these ranges for different soils, particularly in the PNW’s huge diversity of soils and cropping systems. We also need to better understand which indicator measurements are most meaningful and useful in different situations (e.g. this paper by Morrow et al., 2016). If you have a limp, will your blood pressure tell me what I need to know about what is wrong or do I need to do more diagnostic tests? And when during the year should measurements be done? Like any living system, soils are dynamic, and some of the soil properties we measure are constantly changing in response to the environment. Still, these measurements are a helpful guide in understanding the relative functioning of different soils.
Figure 1. Soils with differing history of organic matter inputs are subjected to the slake test (A, above) and the t-shirt decomposition test (B, below). These inexpensive tests provide a quick way to get a sense of the stability and activity of your soil. (Photos by Deirdre Griffin LaHue)
What are some of these indicators?
Let’s go more in depth on a couple of measurements that have become more common in soil health testing and are starting to be offered by commercial test labs: permanganate-oxidizable carbon (POXC) and mineralizable C. These are measurements of “active C” and are categorized by the Cornell Assessment of Soil Health (CASH) and the Soil Health Institute as biological indicators as they are chemical measurements of biological activity, or the potential for biological activity.
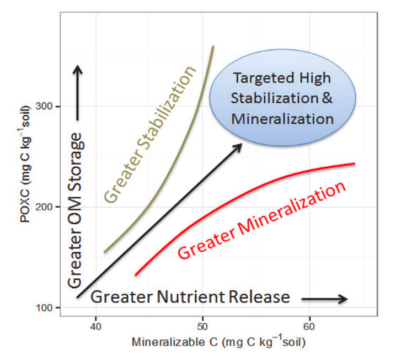
Figure 2. From Hurisso et al. 2016
When soil microbes break down organic matter, they use it as a source of electrons (or energy), oxidizing the carbon molecules in the process. The POXC method uses a chemical oxidizer (potassium permanganate) to mimic the activity of microbes, taking electrons from whatever carbon is available within a set period of time (Weil et al., 2003). Carbon compounds that are easily accessed by the chemical oxidizer are defined as having potential to be “active carbon” while compounds that are protected inside soil aggregates, for example, are not. Soil organisms also release CO2 when they break down organic matter (mineralizing it as they turn it from an organic form to an inorganic form), and this CO2 is another chemical indicator of potential biological activity that we can measure. Though both are indicators of biologically active C, POXC has been associated with greater potential for organic matter stabilization and storage, while mineralizable C is associated with nutrient release (Figure 2; Hurisso et al., 2016).
POXC and mineralizable C have become more common in soil health work as our understanding of the importance of labile, or easily decomposable, carbon has evolved. As mentioned above, soil organisms drive many of the functions we look for in healthy soils, and these organisms need to be fed. Therefore, we now understand that it is not just the total stock of soil organic matter (SOM, which is ~50% C) that is important, but how much of that is C flowing through soil biota (Lehman and Kleber, 2015; See Collins and McGuire, 2019 for more on this).
These active C pools tend to be more responsive to management changes in a shorter period of time (Culman et al., 2012), while total SOM can take years to show measurable differences. They also show seasonal dynamics as the amount of active C from plant root exudates or microbial biomass ebbs and flows (Figure 3 from Culman et al., 2013). There is still work being done to determine when is the best time to sample for these measurements.
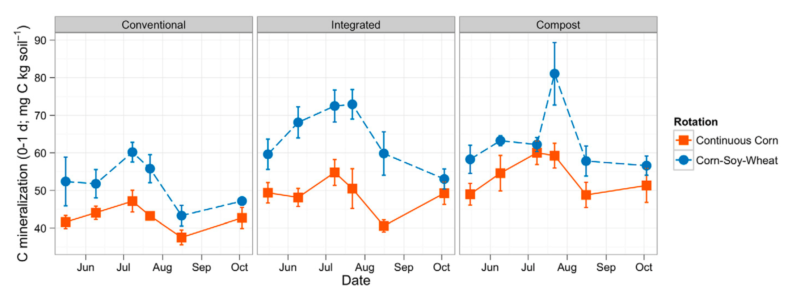
Figure 3. From Culman et al., 2013, showing seasonal dynamics of active C and treatment differences between rotations (continuous corn vs. corn-soy-wheat) and fertility practices (“conventional” vs. “integrated” vs. “compost”)
How do we improve the health of our soils?
At the risk of overusing this metaphor—we know that we can usually keep our bodies healthy by following a few general principles: getting regular exercise, eating nutritious foods, and avoiding destructive activities. However, our exercise routines or diet habits vary from person to person. Similarly, not every land manager can adopt the same practices into their management regime. But we can follow some general principles that seem to move us in the right direction: 1) feeding the soil with some form of organic matter, be it crop residues, green manures, or compost (Oldfield et al., 2018); 2) reducing soil disturbance, particularly when the soil is very wet or very dry and soil structure is more fragile (Zuber et al., 2016; Nunes et al., 2020); 3) having well-managed living roots and soil cover on the ground as much as possible (Marcillo and Miguez, 2017; Kim et al., 2020).
For each system, we must ask: What is the main challenge we need to combat? Which ecosystem services are most critical? How do we value the services that may be provided by increasing soil health?
The popularization of soil health has facilitated a renewed focus on soils as a central part of our ecosystems. Several US states have or are working to pass Soil Health Initiatives, including Washington and Oregon (Figure 4). Ideas of a Federal Soil Protection Act have been discussed among prominent soil scientists. The Maori of New Zealand have elevated soils to personhood status and are using this value system to promote a soil resource management framework that can be applied more broadly. Many recognize that soil conservation plays a critical role in combating climate change and food insecurity globally, and I am hopeful that we can continue to work toward finding economically feasible approaches to improve the health of our soils and value the societal benefits that are gained.
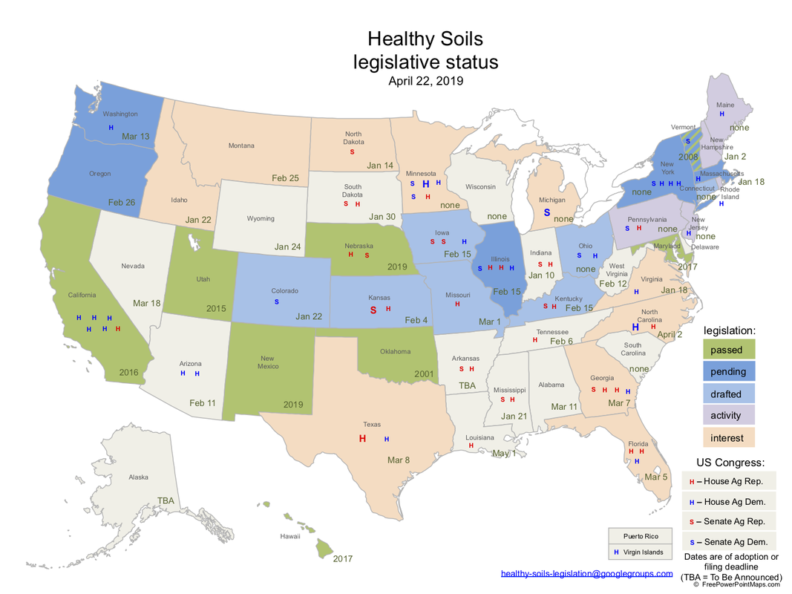
Figure 4. Map showing Heathy Soils legislation across the US (from Soil4climate.org, https://www.soil4climate.org/news/category/healthy-soils-legislation)
Literature Cited
Collins, D., and A.M. McGuire. 2019. Understanding and Measuring Organic Matter in Soil. Washington State University Extension Publication. EM118E.
Culman, S.W., S.S. Snapp, M. A. Freeman, M.E. Schipanski, J. Beniston, et al. 2012. Permanganate Oxidizable Carbon Reflects a Processed Soil Fraction that is Sensitive to Management. Soil Sci. Soc. Am. J. 76(2): 494–504. doi: 10.2136/sssaj2011.0286.
Culman, S.W., S.S. Snapp, J.M. Green, and L.E. Gentry. 2013. Short- and long-term labile soil carbon and nitrogen dynamics reflect management and predict corn agronomic performance. Agron. J. 105(2): 493–502. doi: 10.2134/agronj2012.0382.
Hurisso, T.T., S.W. Culman, W.R. Horwath, J. Wade, D. Cass, et al. 2016. Comparison of Permanganate-Oxidizable Carbon and Mineralizable Carbon for Assessment of Organic Matter Stabilization and Mineralization. Soil Sci. Soc. Am. J. doi: 10.2136/sssaj2016.04.0106.
Kim, N., M.C. Zabaloy, K. Guan, and M.B. Villamil. 2020. Do cover crops benefit soil microbiome? A meta-analysis of current research. Soil Biol. Biochem. 142: 107701. doi: 10.1016/j.soilbio.2019.107701.
Lehmann, J., and M. Kleber. 2015. The contentious nature of soil organic matter. Nature 528: 60–68. doi: 10.1038/nature16069.
Marcillo, G.S., and F.E. Miguez. 2017. Corn yield response to winter cover crops: An updated meta-analysis. J. Soil Water Conserv. 72(3): 226–239. doi: 10.2489/jswc.72.3.226.
Morrow, J.G., D.R. Huggins, L.A. Carpenter-Boggs, and J.P. Reganold. 2016. Evaluating measures to assess soil health in long-term agroecosystem trials. Soil Sci. Soc. Am. J. 80(2): 450–462. doi: 10.2136/sssaj2015.08.0308.
Nunes, M.R., D.L. Karlen, K.S. Veum, T.B. Moorman, and C.A. Cambardella. 2020. Biological soil health indicators respond to tillage intensity: A US meta-analysis. Geoderma 369 (February): 114335. doi: 10.1016/j.geoderma.2020.114335.
Oldfield, E.E., S.A. Wood, and M.A. Bradford. 2018. Direct effects of soil organic matter on productivity mirror those observed with organic amendments. Plant Soil 423(1–2): 363–373. doi: 10.1007/s11104-017-3513-5.
Weil, R.R., K.R. Islam, M. a Stine, J.B. Gruver, and S.E. Samson-Liebig. 2003. Estimating active carbon for soil quality assessment: A simplified method for laboratory and field use. Am. J. Altern. Agric. 18(1): 3–17. doi: 10.1079/ajaa2003003.
Zuber, S.M., and M.B. Villamil. 2016. Meta-analysis approach to assess effect of tillage on microbial biomass and enzyme activities. Soil Biol. Biochem. 97: 176–187. doi: 10.1016/j.soilbio.2016.03.011.
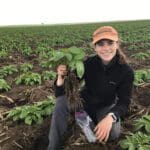
Deirdre Griffin LaHue
Deirdre is an Assistant Professor of Soil Health and Sustainable Soil Management at Washington State University. Her research and extension program focuses on the impacts of agricultural practices on soil health, microbial communities, and the functions they provide.
This article was published by the Washington Soil Health Initiative. For more information, visit wasoilhealth.org. To have these posts delivered straight to your inbox, subscribe to the WaSHI newsletter. To find a soil science technical service provider, visit the Washington State University Extension website or the Washington State Conservation District website.